Circular 694B
John Idowu, Rajan Ghimire, Robert Flynn, and Amy Ganguli
College of Agricultural, Consumer and Environmental Sciences, New Mexico State University
Respectively, Extension Agronomist, Department of Extension Plant Sciences; Agronomist/Assistant Professor, Agricultural Science Center at Clovis; Extension Agronomist, Department of Extension Plant Sciences; and Associate Professor, Department of Animal and Range Sciences, New Mexico State University. (Print Friendly PDF)
Introduction
Soil health or soil quality is a concept that has increased in popularity over the past several years, especially since the early 1990s. It continues to gain traction among farming and ranching communities, soil managers, scientists, agricultural Extension specialists, and other groups that work with soil. “Soil health” is an important focus for many agricultural groups interested in regenerative and sustainable crop and livestock production as well as land management. While awareness of soil health is increasing, it is important to have a good understanding of what soil health entails, how it is measured, and how to manage it for optimal and sustainable delivery of the ecosystem services that soils provide.
What is Soil Health?
To define soil health appropriately, we need to consider the words that form “soil health.” “Soil” is defined by the Soil Science Society of America (SSSA) as “the unconsolidated mineral or organic material on the immediate surface of the earth that serves as a natural medium for the growth of land plants.” “Health” is defined by Merriam-Webster as “the condition of being sound in body, mind, or spirit.” We can restructure this definition of health to apply to the soil. Combined with the SSSA’s definition of soil, “soil health” can be defined as “the state of the soil being in sound physical, chemical, and biological condition, having the capability to sustain the growth and development of land plants.”
There are many definitions of soil health; however, emphasis is always placed on the sustained ability of soil to provide services, such as production of crops and other agricultural products, retention and filtration of water, habitat for diverse organisms, and recycling of nutrients. Incorporating all these elements is a holistic definition of soil health as “the capacity of soil to function as a vital living system, within ecosystem and land-use boundaries, to sustain plant and animal productivity, maintain or enhance water and air quality, and promote plant and animal health” (Doran and Zeiss, 2000).
Soil Components
To appreciate the complexity of soil health in different agricultural systems, we have to learn about the components of the soil. Soil is composed of solid particles, developed through the soil-forming processes. These particles have different shapes and sizes, and are classified as sand, silt, or clay based on their size. Sand particles are the largest (0.05–2 mm), followed by silt (0.002–0.05 mm), while clay particles are the smallest (<0.002 mm). In between these particles, soils have pore spaces that can be filled with air and/or water, depending on the state of the soil (Figure 1). Soils with more sand will have larger pores compared to fine-textured or “heavy” soils, which will have smaller particles and smaller pores. The relative composition of sand, silt, and clay determines soil texture (Figure 2).
Figure 1. Components of the soil, including mineral particles, water, air, and the soil organic matter.
Figure 2. Soil textural triangle used for determining soil texture if the percentages of sand, silt, and clay are known (source: USDA Natural Resources Conservation Service).
Soils have different textures based on the proportion of different-sized particles measured after removing all the rocks, cobbles, and gravel larger than 2 mm. Textures range from sand, which has predominantly sand-sized particles, to clay, which has high percentages of very small particles. The soil particles make up about 45% of the volume of soil (Figure 1).
A soil that is saturated with water will have no air in the pore spaces, while a soil that is dried out will have predominantly air in the pore spaces. The total pore spaces are about 50% in a soil with a very good structure (Figure 1). In an ideal cropping soil, the remaining 5% of soil would be made up of the soil organic matter, which is biological material at various stages of decomposition (Figure 1). New Mexico soils typically have 1–3% soil organic matter, but even though this fraction is small compared to the other soil components, it is critical for soil functions, and a goal should be to increase this amount. Organic matter provides the food for soil organisms that regulate nutrient availability to plants and many other soil functions that maintain or improve land productivity.
Managing soil health means implementing practices that either maintain or enhance the soil’s physical, chemical, and biological attributes to improve soil functions. The functioning of the physical, chemical, and biological aspects of the soil is not mutually exclusive. They operate synergistically and interact in a complex way to deliver specific soil services and to enhance ecosystem functions, such as nutrient availability, erosion control, and water infiltration. A way to visualize sustainable productivity in relation to soil health is to think of a wheel, where the hub of soil health relies on the spokes of the soil’s physical, chemical, and biological functions operating in synergy to drive sustainable productivity (Figure 3).
Figure 3. Soil health is connected to sustainable soil productivity through balanced physical, chemical, and biological soil attributes.
Soil Properties
Physical
Soil physical properties are mainly controlled by the soil matrix. One of the most important soil physical properties is the soil texture, which was described in the section on soil components. Not all soils are created equal. A variety of soil textures can be found in agricultural lands depending on the relative composition of different soil particles (sand, silt, and clay). Soil texture is not an attribute that you can easily change. However, every type of soil texture can be managed for sustainable productivity. Texture influences many soil functions, such as how fast water will flow within soil, how much water the soil can hold, and how fertile the soil will be. For example, soils with a high percentage of clay will drain water slowly compared to soils with a high sand percentage. Clay soils generally hold water tightly because the soil pores are smaller, while sandy soils will retain little water due to large soil pores.
Another physical property that is important for production agriculture is the bulk density, which is a measure of how closely the soil particles are packed together. If soil particles are packed too closely together, that soil is a compacted soil. Compacted soils will inhibit plant rooting and water movement in the soil. You can read more about soil compaction and management strategies in NMSU Extension Circular 672, Understanding and Managing Soil Compaction in Agricultural Fields (https://pubs.nmsu.edu/_circulars/CR672.pdf).
Soil structure is also an important soil physical property that affects many functions within the soil. Soil structure will affect water movement, resistance to erosion, and nutrient cycling. When studying soil structure, we usually look at the distribution and stability of soil aggregates within the soil. Soil particles normally stick together in lumps of different shapes and sizes called aggregates. The distribution of these aggregates in terms of size affects the soil’s ability to withstand wind/water erosion and its pore size distribution. The stability of soil aggregates is critical to soil function. Unstable aggregates at the surface of the soil can easily be broken down by raindrops, sealing the pores at the soil surface (Figure 4). When the surface pores are sealed, water entry into the soil will be drastically reduced. The leftover water that cannot enter the soil will then travel on the soil surface, moving off to other areas in a process called runoff. Water movement across bare surfaces carries soil particles, causing tons of soil to move from one place to another. This process is called soil erosion. When erosion occurs, soil particles, dissolved nutrients, and organic matter particles are removed from agricultural fields, leading to lost fertility and depletion of organic matter.
Figure 4. USDA Natural Resources Conservation Service demonstration of soil erosion where removal of plant cover leads to increased water runoff and soil loss through reduced infiltration. Runoff is collected in the front bottle under the soil boxes; soil boxes 1, 2, and 4 have no surface cover and more runoff compared to boxes 3 and 5, which have standing plants and litter.
Chemical
Chemical properties of soil are related to properties that directly affect plant nutrition. Plants need an adequate supply of nutrients to grow and complete their reproductive phases. If any of the essential nutrients are not available, the plants will not grow well or may even die. On the other hand, some nutrients can cause problems for plants if their quantities in the soil are too high.
There are 17 nutrient elements that most land plants need for productive growth and development (Epstein and Bloom, 2005): carbon, oxygen, hydrogen, nitrogen, phosphorus, potassium, calcium, magnesium, sulfur, zinc, manganese, boron, copper, chlorine, iron, nickel, and molybdenum. Carbon, oxygen, and hydrogen are often not a concern since these nutrients are obtained through the air and soil water. Elements of concern for soil fertility management are classified into three categories. The first category is called primary nutrients: nitrogen, phosphorus, and potassium. These primary nutrients are needed by plants in relatively large amounts. The second category is called secondary nutrients: calcium, magnesium, and sulfur. These nutrients are needed in substantial amounts, but much less than the primary nutrients. The third category is called micronutrients: zinc, manganese, boron, copper, chlorine, iron, nickel, and molybdenum. They are needed in small quantities, but they play a large role in plant growth and development. The classification of nutrients does not indicate their usefulness to plants. All these nutrient elements are essential for plants, and the absence of any of them will cause plant disorders and affect plant growth and yield.
Other soil chemical conditions that affect crops include soil pH, salinity, and the sodium adsorption ratio (SAR). Soil pH can affect nutrient availability in the soil. It is measured on a scale from 1–14. A pH of 7 is regarded as neutral, a pH below 7 moves the soil toward the acidic region, and a pH above 7 moves the soil toward the alkaline region. The optimal pH for soil nutrient absorption is between 6.5 and 7.5. Within this range, most soil nutrient elements will be available for crop uptake. However, below pH 6.5 or above pH 7.5, certain elements will become less available, even if they are present in the soil. That is why soil fertilizers need to be applied to some soils to compensate for lower availability of a nutrient due to pH issues. To learn more about soil nutrients and pH, please see NMSU Extension Circular 676, Interpreting Soil Tests: Unlock the Secrets of Your Soil (https://pubs.nmsu.edu/_circulars/CR676.pdf).
Salinity and sodium problems are other issues that can occur in agricultural soils of New Mexico. Salinity is the accumulation of excess salts within the rooting depth of plants. Excess salts in the soil can lead to plant disorders and eventual death if not well managed. Sodium problems occur when the amount of exchangeable sodium in the soil is too high relative to calcium and magnesium ions. In soil with sodium problems, the SAR will be high (>13) and such soils will lose structural stability due to a breakdown of soil aggregates. When this happens, water cannot move into and through the soil. At times, there can be a combination of salinity and sodium problems in the same soil. To learn more about salinity and sodium problems and the management strategies that are available for soils in New Mexico, see NMSU Extension Circular 656, An Introduction to Soil Salinity and Sodium Issues in New Mexico (https://pubs.nmsu.edu/_circulars/CR656.pdf). Another resource is the NMSU Western Soils website (westernsoil.nmsu.edu).
Biological
The biological component of the soil is perhaps the most intriguing attribute because it comprises both the living organisms and the dead organic materials they feed upon. It is important to note that bacteria, fungi, and many other living organisms in the soil, in association with living plants and dead organic matter, are the key drivers that maintain and even regenerate healthy soil functions. The soil food web is extremely complex, with different classes of organisms occupying different trophic (feeding) levels (Scheu, 2002). There are five trophic levels defined for the soil food web that transform nutrients from one form to another (Figure 5).
Figure 5. The soil food web describing the various feeding relationships of the organisms in the soil (source: USDA Natural Resources Conservation Service).
At the bottom of the food web (Level 1) are materials produced from photosynthesis, such as dead plant parts that become organic matter that helps feed the soil communities. Root exudates also belong to this category since they are important in feeding microbial populations. Other materials that belong to Level 1 include animal waste products like manures and artificially created materials, such as composts, biochar, and many other biologically based soil amendments, all of which can be added to enrich the soil. The organic compounds found in plants, organic amendments, and waste byproducts supply energy and carbon for most of the soil organisms and provide a foundation for healthy soils.
The next level (Level 2) includes decomposers, such as bacteria, fungi, nematodes, and several other microscopic organisms, all of which are actively breaking down the organic materials in the soil. This decomposition causes nutrients contained in the organic materials to be released as elements that plants can use for growth and development. This process is called mineralization of the organic matter, where organic compounds are converted to forms that the plant roots can use. Even a single teaspoon of soil harbors billions of bacterial cells, millions of fungal cells, and thousands to millions of other organisms that decompose organic residues and supply nutrients. Without these decomposers, it is difficult for organic matter to be effectively recycled within the soil.
Level 2 also includes mutualists, pathogens, parasites, and root feeders, all of which can be present in the soil. Mutualists are organisms that have symbiotic, or mutually beneficial, relationships with plants, such as the mycorrhizal fungi that associate with the roots of many plants and help them to improve nutrient and water uptake. Additionally, rhizobium bacteria in soil are involved with nitrogen fixation in the root nodules of legumes (see NMSU Extension Guide A-129, Nitrogen Fixation by Legumes [https://pubs.nmsu.edu/_a/A129.pdf]). Pathogens, parasites, and root feeders are disease-causing organisms in soil that can affect plant productivity. Part of good soil health practices involves minimizing these disease-causing soil organisms while also maximizing beneficial soil organisms.
Level 3 of the soil food web includes grazers, shredders, and predators. Some of the organisms at this level are small arthropods (insects), larger nematodes, and protozoa, which feed on smaller microorganisms. Also, at this level are several organisms involved in shredding and feeding on the soil organic materials. An important example of this type of soil organism is the earthworm. Earthworms perform very important functions in agricultural soils, feeding on dead and decomposing organic materials and dragging them from the soil surface into deeper soil layers. As these organic materials pass through the earthworms’ intestines, they become fragmented, are mixed with soil particles, and are further broken down in their guts before being excreted as waste products in the form of casts. Because of the high amount of organic matter in earthworm casts, they are usually stable against raindrop impacts and are rich in nutrients compared to the surrounding soil. Apart from nutrient cycling, earthworms can improve soil water infiltration and retention as they create channels in the soil due to their upward and downward movement while finding organic materials to feed upon.
Levels 4 and 5 of the soil food web are mostly composed of higher-level predators. Level 4 predators are smaller, including several insects and nematodes that actively feed on organisms lower in the soil food web. Level 5 predators are composed mostly of larger vertebrate animals that feed on soil insects, worms, and other invertebrates.
The two most important components that enable effective functioning of the soil biological systems are soil organic matter and living roots in the soil. As discussed earlier, organic materials produced by photosynthesizing plants occupy the lowest level of the food web. Without these materials, the whole food web structure would break down. For example, due to the lack of organic material in beach sands, the activity of soil organisms is minimal.
Organic materials in agricultural soils are at various stages of decomposition due to diverse organisms that are involved in the process. Depending on the state of decomposition, organic materials in the soil are classified into three broad categories: “the living,” “the dead,” and “the very dead” (Magdoff and Van Es, 2000). “The living” component consists of roots that are still alive and microbes and other soil organisms that are still functional in the soil. “The dead” component consists of recently shed plant parts like dead roots and leaves, manure, compost, and other biological materials added to the soil. The dead component can also be viewed as the active fraction of soil organic matter since these materials provide food for soil organisms to increase their rapid growth and population numbers in the soil. “The very dead” component is the product of organic matter decomposition that remains after the organic materials have undergone initial, rapid breakdown. Further decomposition is extremely slow because the material is so stable and resistant to further breakdown. The material that accumulates in the soil at this stage is called humus or humic substances. Unlike fresh plant residues, humus can absorb tremendous amounts of water (up to 20 times its weight; Stevenson, 1994) and protects several plant nutrients, such as potassium, magnesium, and calcium, against leaching losses.
In addition to soil organic matter, soil organisms need plant roots to thrive. Most soil microorganisms are found near the plant roots in a region called the rhizosphere. There are 1,000–2,000 times more microorganisms associated with plant roots in the rhizosphere compared to the soils beyond this zone. Microbes are so abundant in rhizospheres due to the simple carbohydrates in root exudates that the plants produce, which microbes can use as food. An average of about 35% of the products formed during plant photosynthesis are released back into the soil as root exudates to stimulate microbial growth within the soil. Diverse plant species, like those found in rangelands or different cover crops planted in croplands, have diverse rooting depths, which support microbial diversity throughout the soil profile. That is why the presence of living roots is essential for maintaining good soil health.
Why Should We Care About Soil Health?
Soil is one of the natural resources that supports human civilization. Without a productive and healthy soil, the prospect of producing enough food to feed an ever-increasing human population is impossible. We have many degraded soils across the globe that are no longer productive and can only be regenerated to a fruitful state by applying soil health principles. The global trends show that 20% of cropland, 16% of forest land, 19% of grassland, and 27% of rangeland are in persistent decline in productivity due to soil degradation (UNCCD, 2017). This can have significant effects on global food security if not addressed.
In New Mexico, a major effect of soil degradation is the high rate of soil erosion by wind. Additionally, water erosion can become a problem during the monsoon period (July–September) due to high-intensity, short-duration rainfall that is typical for our region. These high-intensity rainfall events can lead to ephemeral (temporary) or permanent gully formations in both range and farmlands (Figure 6). However, while tillage in cropland may correct small ephemeral gullies, permanent gullies may form in rangelands if corrective action is not implemented.
Figure 6. A scoured field after a large rain event in 2018 (photo by Houston McKensie).
The rate of soil erosion by wind has increased in New Mexico from about 16 tons/acre in 1982 to 23 tons/acre in 2015 (USDA, 2018). This rate of soil erosion is far higher than the tolerable soil erosion rate of 1–5 tons/acre per year. We are losing soil from crop and rangelands at a rate that exceeds the rate at which soil formation occurs. Through wind and water erosion, we are losing not only soil mineral particles but also the more valuable soil organic matter. We are losing both the fertility and the water-holding capacity of the soil, thus degrading soil health. A study conducted in New Mexico found that wind erosion removed 25% of the total organic carbon and total nitrogen from the top two inches of a bare soil over three windy seasons (Li et al., 2007). Another study in eastern New Mexico showed that cultivated soils in that region have lost nearly half of their soil organic matter that would have been otherwise stored in native grasslands (Thapa et al., 2018).
While soil can be lost quickly through erosion, soil formation is a very slow process. It may take between 100 to 10,000 years for an inch of soil to form, depending on the soil-forming processes that occur in a given region. This slow rate of formation makes soil a finite resource if it is mismanaged since it is a slowly renewable natural resource. An unhealthy soil will be prone to loses by wind and water erosion. Therefore, developing and maintaining healthy soils is necessary to ensure long-term soil productivity and food security.
Another reason we should care about soil health is the environmental, economic, and public health consequences arising from degraded and unhealthy soils. For example, when dust storms develop, suspended sediments have been proven to be hazardous for road safety and constitute serious health issues for those with respiratory problems. Also, sediments that are transported away by erosion often accumulate in places where they are not wanted, requiring extra costs to remove the deposited sediments (Figures 7 and 8). Poorly managed soils can also lead to the contamination of surface water and groundwater due to agrichemicals attached to the dust and soil.
Figure 7. Dune formation from unprotected soil (photo by Patrick Kircher).
Figure 8. Image from Google Earth over Roosevelt County showing soil deposition in the field after a major wind erosion event.
How Do We Measure Soil Health?
There is no direct method to judge the status of soil health. Just like human health, there is no single machine that can be used to assess whether a person is healthy or not. However, just as we can take different measurements to judge a person’s health, it is also possible to measure specific soil properties to detect whether a soil is healthy or not. Such measurements may be quantitative or qualitative, or a combination of both.
As described earlier, soils include physical, chemical, and biological aspects. In order to develop the best measurements for estimating soil health, we must look at the three aspects and select specific measurements that relate to our intended use of the soil. The best set of measurements that will allow us to judge the status of soil health is called the minimum data set (Rezaei et al., 2006). The measurements that make up the minimum data set are called soil quality indicators or soil health indicators. The first step in soil health assessment is to identify suitable indicators (measurements) that will constitute the minimum data set.
Selecting the required minimum data set of soil health indicators is not an easy task. It is often based on years of experimentation and sample analyses for multiple soil measurements under different production systems in order to identify which measurements are most sensitive to changes in management practices. The purpose of soil health assessment is to allow a farmer, rancher, or land manager to know in which direction the soil health is going—for better or for worse. When we know the direction of change, we can implement specific management practices to mitigate degradation if the soil is in poor shape or continue the current practices if the soil is improving. Without knowing the directional changes in soil health, it is not possible to effectively plan and implement better management practices.
The choice of soil health indicators for assessment often varies with the intended use of the soil. For example, the set of soil measurements used to assess the soil for sustainable rangeland may be different from the measurements used for sustainable crop production. Therefore, there are no universal soil health indicators that will function for all systems. Part of soil health assessment research is often focused on identifying different indicators that will best suit different agroecosystems.
Many soil health assessment protocols have been developed around the country, but it is important to realize that a soil health assessment protocol that was developed in one region may not be suitable for another. The best soil health assessment protocols for a given region are those that were developed specifically within the agroecosystems of that region. For example, soil health assessment protocols developed in the humid, temperate region of Illinois may perform poorly in the arid and semi-arid agroecosystems of New Mexico; soil and crop management issues differ between both regions.
Table 1. Soil Measurements Identified for Quantitative Soil Health Assessment in Arid and Semi-arid Agroecosystems |
||
Soil Physical Indicators |
Soil Chemical Indicators |
Soil Biological Indicators |
Soil compaction level (in field) |
Soil primary nutrients (N, P, K) |
Soil organic matter |
Soil bulk density (in field) |
Soil micronutrients (Zn and Fe) |
Permanganate oxidizable carbon |
Dry aggregate size distribution |
Soil salinity |
Nitrogen mineralization |
Wet aggregate stability |
Soil sodicity (sodium problem) |
Carbon mineralization |
Soil texture |
Soil pH |
Total microbial biomass |
Available water capacity |
Microbial diversity index |
Within New Mexico, soil and cropping system scientists have been working for several years to identify relevant soil health indicators suitable for production agriculture in the region, some of which are listed in Table 1. Some of these indicators include measurements that are taken directly in the field, while others are laboratory measurements performed on soil samples collected from the field. For rangeland systems, the widely used guide “Interpreting Indicators of Rangeland Health” (Pellant et al., 2018) provides qualitative and quantitative assessments of a site’s biological integrity, soil stability, and water movement into the soil. A more complete assessment of soil health requires the same laboratory assessments mentioned above.
To start with quantitative soil health assessment of your farm fields or rangelands, contact your local NMSU Extension agent (https://aces.nmsu.edu/county/), Soil and Water Conservation District, or NRCS office to seek further guidance and assistance.
Apart from the quantitative soil health assessment, there are also qualitative measures that can be used in the field to monitor the state of your soil health. The “In-Field Soil Health Assessment Worksheet,” currently being developed by the New Mexico NRCS, provides useful field-based observational measurements for farmers and soil managers. The in-field assessment includes observations such as soil cover, residue breakdown, surface biological and physical crusts, water ponding, compaction measurement, water-stable aggregates, soil structure, soil color, plant roots, biopores (Figure 9), and biological diversity (https://www.nrcs.usda.gov/wps/portal/nrcs/detailfull/soils/health/biology/). All these measurements are rated in the field on a worksheet to help identify areas of soil constraints. Based on the issues identified, the land manager can modify or select appropriate management practices to address the constraints.
Figure 9. Presence of earthworms and biopores in soil as indicators of soil health (photo by Rajan Ghimire).
Qualitative approaches are useful for short-term management and educational purposes to help land managers understand the processes taking place in their soils. However, for long-term soil health management, quantitative assessment protocols are necessary to precisely understand the direction and magnitude of soil change.
How Do We Manage Soil Health?
As emphasized in previous sections, the purpose of a soil health assessment is to manage the soil for sustainable production and to achieve sustainable productivity of the soil. Figure 10 illustrates several strategies that can be used. These strategies include:
- Reduce soil disturbance (farmlands and rangelands)
- Crop rotation practices (farmlands)
- Cover cropping practices to promote plant diversity and living roots (farmlands)
- Diversify production systems (farmlands and rangelands)
- Add soil organic amendments (farmlands and rangelands)
- Integrate livestock into cropping systems (farmlands)
- Promote diverse plant species with different rooting depths (rangelands)
- Sustainable animal grazing practices (rangelands)
- Be patient for the results and don’t expect positive change overnight (farmlands and rangelands)
Figure 10. Management strategies that lead to soil health maintenance and improvement.
1. Reduce soil disturbance: Intensive soil tillage is one of the greatest catalysts for soil degradation. Although plow tillage systems are a longstanding practice for land preparation in many places, they are the most detrimental practice to soil health in both the long and short term. Breaking up the soil and intensively working it with tillage equipment can lead to many problems. Tillage is highly destructive because it disrupts the habitats and populations of soil microorganisms that contribute significantly to maintaining and improving soil health. One of the major problems that modern intensive tillage has caused is the rapid loss of the soil organic matter, which is the food for microbes and a binding agent for soil aggregates.
The more the soil is tilled, the higher the rate of organic matter oxidation (organic matter loss) in which soil organic carbon is converted to carbon dioxide and lost to the atmosphere. Therefore, an intensively tilled soil will likely have poor soil health because of the low organic matter content. This is true for many of the arable soils in New Mexico. Using reduced tillage or conservation tillage methods will preserve and improve the soil organic matter over time and will be less disruptive for the soil microorganisms. Many research studies are now showing that reduced tillage methods, such as strip tillage and no tillage, can produce similar crop yields as the conventional plow-till method in arid and semi-arid farmlands (Darapuneni et al., 2019; Idowu et al., 2019). This means that by transitioning to reduced tillage practices, crop producers can remain profitable in terms of yield and at the same time conserve and improve the soil health of their fields. An added benefit to reduced tillage is fewer field passes for land preparation, leading to savings in fuel and tillage costs.
Reducing tillage for crop production is not an easy task. There are multiple challenges that farmers must overcome before achieving effective reduced tillage benefits. However, with proper information and guidance, it is possible to engage in successful conservation tillage practices in arid and semi-arid cropping systems. To learn more about reduced tillage, please see NMSU Extension Guide A-152, Reducing Tillage in Arid and Semi-arid Cropping Systems: An Overview (https://pubs.nmsu.edu/_a/A152.pdf)
Reducing soil disturbance is important in rangelands for the same reasons. Soil disturbance in rangeland systems typically comes from practices such as intense livestock overgrazing, undergrazing, mechanical treatments like roller chopping and chaining, rangeland seeding using conventional seeders versus minimum-till seeders, and subsoil plowing.
2. Crop rotation practices: Crop rotation is a method of farming in which farmers grow crops from different plant families in a well-planned sequence over multiple seasons in the same field. For example, a farmer may grow alfalfa for three years, after which chile peppers are grown for two years and then cotton for another two years before growing alfalfa again. In this case, the farmer has a seven-year rotation cycle. These three crops–alfalfa, chile, and cotton–belong to different crop families. Crop rotation can be of any length depending on farmers’ preferences and other conditions on the farm, or local conservatory regulations. Cover crops also count within any rotation cycle. The key factor in rotation is that a variety of crops belonging to different families are being grown in the same soil.
There are several benefits crop rotation can bring to any farming system. An important benefit of crop rotation is the ability to break the disease cycle in a given field. Growing the same crop continuously in the same soil often leads to the buildup of disease-causing organisms called pathogens. If rotations are not practiced, these pathogens can multiply in the soil and exceed the threshold population for causing plant diseases. When rotations are practiced by growing a plant belonging to another family, the pathogen cycle becomes disrupted because the new crop belonging to a different family cannot serve as a host for the pathogen. Therefore, the pathogen population will be reduced rapidly in the soil.
Also, by growing different crops the microbial population and diversity in the soil are enhanced (Vukicevich et al., 2016). There are different microbes that associate with different plant roots (Berendsen et al., 2012). The greater the diversity of crops you grow on your land, the greater the diversity of soil microbiomes you will have, resulting in better soil health.
3. Cover cropping practices: Cover crops are plants that are grown in between cash crop cycles to protect the land from erosion and to add root exudates and biomass for soil improvement. Cover crop biomass must be returned to the soil after the desired growing period for the soil health benefit to be fully realized. If the cover crop is harvested off the field as a forage crop, the soil health improvement derived from the cover crop may be undetectable. Soils of the arid and semi-arid Southwest have very low organic matter contents; therefore, growing a cover crop is one way to increase the soil organic matter through the addition of biomass to the soil.
Some cover crops deliver other benefits to the soil. For example, legumes have the capacity to fix nitrogen in the soil by associating with a type of bacteria called rhizobium. Rhizobium can convert atmospheric nitrogen to plant-useable nitrogen. When leguminous cover crops are worked into the soil, the nitrogen that was fixed and translocated into the plant is made available for the subsequent crop after residue decomposition. This means that the external nitrogen demand of the cash crop following the legume cover crop will be reduced. NMSU Extension Guides A-129, Nitrogen Fixation by Legumes (https://pubs.nmsu.edu/_a/A129.pdf), and A-130, Inoculation of Legumes (https://pubs.nmsu.edu/_a/A130.pdf), explain this process.
A crop family that can be used to control soil-borne diseases is the brassica (mustard) family. Plants in the brassica family contain compounds called glucosinolates that can suppress soil-borne pests, such as disease pathogens, parasitic nematodes, and weed seed germination (Sarwar et al., 1998). If you are interested in learning more about cover crops in arid and semi-arid agricultural systems, please see NMSU Extension Guide A-150, Principles of Cover Crops in Arid and Semi-arid Farming Systems (https://pubs.nmsu.edu/_a/A150.pdf).
4. Diversify production systems: Introducing diversity into your production system will allow you to build a healthy soil. Diversifying your production system can be done in different ways. The key factor is to intentionally increase biodiversity on the farm or range either by increasing the number of crop species planted or by integrating livestock into the cropping system. Diversified farming systems include practices such as mixed varieties and mixed cropping, integrating livestock into crop production, cover cropping, crop rotation, fallowing fields, hedgerows, buffer strips, and many other practices (Kremen et al., 2012). All these practices introduce new species into the farming system that can improve soil health and support agricultural biodiversity.
Other benefits of diversified farming systems include improvements in nutrient cycling, soil water management, pest control, and habitats for pollinators. A study conducted in eastern New Mexico, in which animal grazing was integrated into crop production systems, led to improvement in soil health through increases in microbial community size, soil organic matter, and total nitrogen compared to conventional cropland (Ghimire et al., 2019). Apart from the soil health improvement, diversifying crop production on a farm can help minimize losses since production risks are spread across different commodities that the farm produces.
5. Add soil organic amendments: Organic amendments are any material of plant or animal origin that can be added to the soil to improve soil conditions and stimulate biodiversity. Examples of amendments include manure, compost, biochar, and similar materials. Long-term research studies have shown that regular addition of organic amendments to the soil can improve soil fertility, soil biological functions, and soil physical characteristics (Diacono and Montemurro, 2011). Adding organic amendments is useful in arid and semi-arid agricultural systems due to the scarcity of vegetation materials that replenish soil organic matter. In most cases, farmers apply organic amendments to supplement crop nutrition on the farm. An additional benefit of adding these amendments is increased microbial activity. Increased microbial activity after soil amendment application breaks down organic materials and releases nutrients.
While growers are encouraged to use organic amendments, the availability of amendments may limit the possibility of using this method for soil health improvement. For example, in order to use dairy manure, the farmer must be able to afford the cost of transporting manure from a dairy to the intended field. If the distance to the source of manure is too far away from the farm, then using manure may not be economically feasible for the farmer.
Another limitation to using organic amendments is the cost of procuring them. If materials have been produced by a third party, such as biochar or compost, they must be purchased. Such organic amendments are often too expensive for farmers unless used in small plots like backyard gardens. Such small soil areas are even more prone to over-application of amendments. Costs can go higher if the amendments must be shipped from far away. When organic amendments are nearby and affordable, they provide an excellent way to improve soil health. However, soil managers must be careful about using some amendments. It is good to know the chemical analysis of the amendment you intend to use and to test your field’s soil according to suggested procedures in NMSU Extension Guide A-146, Appropriate Analyses for New Mexico Soils (https://pubs.nmsu.edu/_a/A146.pdf). For example, some manures may have very high salt content. If they are applied at very high rates, they can cause salinity issues in the soil and adversely affect the crops in the field. This reinforces the need for a laboratory analysis of the organic amendment you want to use, especially if they are coming from other places or you are purchasing them. Alternatively, you can ask the vendor to provide product analysis results. For more help in choosing appropriate organic amendments or interpreting the amendment test results, contact your local Cooperative Extension Service office.
Rangelands in New Mexico are characterized as being extensively managed systems rather than intensively managed systems like croplands or irrigated pasture land. Extensively managed rangeland systems use small inputs of labor, infrastructure, and capital relative to the amount of land used. It is therefore not practical to consider most of these amendments in New Mexico rangelands because the economic and ecological returns will be minimal. In some situations, amendments that drastically change soil chemistry can have negative effects because they favor weedy species that outcompete desirable native species that have evolved to thrive in low-nutrient environments. Just as in croplands, amendments may have unintended consequences in rangelands. Therefore, as a rule, it is essential to know the properties of any amendment that is applied to the soil.
6. Integrate livestock into cropping systems: Livestock are a big part of agriculture in New Mexico. In crop-range-livestock integrated systems, cattle, goats, and sheep are often used to graze crop residues and stubble during the winter season, while crops are grown from spring through the fall. Animals are moved to the rangeland when cash crops are growing in the crop production fields. Integrating livestock into the cropping system through winter grazing can benefit producers by increasing the efficiency of farm resource utilization and decreasing the need for synthetic fertilizers and pesticides, while improving soil health (Ghimire et al., 2013). Livestock-integrated systems support the growth of diverse microbial communities that promote soil organic matter accumulation and the development of healthy soils. Grazing speeds up the decomposition of leftover plant residues, thus enhancing the availability of nutrients like nitrogen, phosphorus, and sulfur. Hoof actions of animals can bring more residue in contact with the soil, while the saliva of the grazing animals helps the growth of microbial decomposers, leading to better soil organic matter formation and nutrient release. However, high-intensity grazing of limited amounts of residue (overgrazing) could negatively impact soil health due to excessive trampling and hoof-induced compaction of the soil surface. Overgrazing can also lead to aggregate breakdown and reduced water infiltration into the soil. Therefore, an optimal balance of crop-range-livestock is critical for maintaining or improving soil health in livestock-integrated systems.
7. Promote diverse plant species with different rooting depths: Introducing species with diverse rooting depths is essential for the long-term sustainability of rangeland soil health. Having a rich diversity of species has been shown to improve soil health due to increases in soil carbon sequestration and soil biodiversity (Chen et al., 2018). Diverse plants produce various soil enzymes that recycle nutrients and increase soil organic matter accumulation. Plant roots also release various compounds that bind or “glue” the soil particles together to form aggregates, which is important for infiltration, water retention, and erosion control. Introducing plant species with diverse rooting depths will also promote nutrient cycling within the rangeland by providing opportunities for plants with deeper roots to capture nutrients that would otherwise be lost to leaching. Increased plant diversity is also associated with better aggregate formation and stability. One of the major indicators used in rangeland health assessments is plant species loss or biotic integrity, which is defined as “the capability of supporting and maintaining a balanced, integrated, adaptive community of organisms having a species composition, diversity, and functional organization comparable to that of the natural habitat of the region” (Pellant et al., 2018).
8. Sustainable animal grazing practices: Grazing strategies in rangeland systems can affect soil health positively or negatively. Many soil properties can be affected by improper stocking density of animals (number of animals per land unit area) that are grazing a given land. One of the major problems encountered due to overstocked grazing is the compaction of agricultural fields and the breakdown of the surface soil structure (Mulholland and Fullen, 1991). With overgrazing, the plant communities being grazed can be rapidly depleted and damaged, preventing their regrowth and thereby limiting the utility of the land for future grazing. Some important factors to consider that can affect soil health when grazing the land include:
a. Stocking species and density. The heavier the animals are, the more impact they have in terms of soil compaction. For example, grazing sheep and goats will cause less compaction than the equivalent number of cattle. Also, the higher the stocking density, the more soil compaction and breakdown of soil structure will occur.
b. Soil moisture affects the predisposition of a soil to compaction and structural breakdown. The wetter the soil, the more compaction and structural breakdown can occur. Therefore, grazing a very wet field will affect soil health negatively by causing compaction and loss of structure.
c. Vegetation cover can provide protection by acting as a barrier between animal hoofs and soil. Vegetation also increases the load-bearing capacity of the soil, thus enhancing its ability to withstand heavy weight without experiencing compaction. The more vegetation on the grazing land, the better the soil will be able to support sustainable grazing. To maintain and improve soil health, the vegetation cover should not be overgrazed, but appropriately grazed to ensure recovery and regrowth of the plant communities in the grazing land.
9. Be patient for the results: Soil health management is a long-term strategy. Just like human health, correcting soil problems cannot happen overnight. It takes consistent and diligent management to address soil health constraints. The following steps are necessary to build an effective long-term soil health management strategy for your land.
a. Education: Land managers need to be well educated on soil health management strategies and how to apply them to their land.
b. Thinking: There is a need to give careful thought to different options that are available to be able to know what will work and what will not work in your farm situation.
c. Planning: After identifying which soil health management strategy you want to use, you need to carefully plan when and how you will implement the practices. For example, if you want to use manure, your planning should include soil and manure testing, figuring out application rates and times, and planning for follow-up soil testing and field monitoring that needs to be done.
d. Discussion and reading: It may be important to speak to someone who has tried the specific soil health practice that you are planning to use. They may be able to share information that will enable you to succeed. You can also discuss options with your local NMSU Cooperative Extension Service agent or specialist to help you make the right decisions. Reading soil health books is a highly effective way to learn more. Some of the books and websites that can help with learning more about soil health are listed after the references section of this publication.
e. May require investment: Most of the time, planning to improve soil health requires some form of investment of either time or money. For example, to use cover crops, you must buy the seeds and pay irrigation costs to establish the cover crop. Although there may not be an immediate return on your investment, it will yield good results in the long term.
Conclusion
Soil health assessment and management will continue to play a prominent role in agricultural production systems of arid and semi-arid agroecosystems. Healthy soil is more resilient against fluctuations in growing conditions. With yearly weather uncertainties, the resiliency of the soil system needs to be enhanced to cope with these variations. Building and improving the soil health of New Mexico crop and rangelands will ensure continued productivity, enhance farmers’ incomes, and promote food security. Building and maintaining a healthy soil is not an easy task in the arid and semi-arid Southwest, especially in the face of droughts and other production challenges. With the resolution of the farming and ranching community, it is possible to adopt soil health practices that will maintain and improve soil productivity.
References
Berendsen, R.L., C.M.J. Pieterse, and P.A.H.M. Bakker. 2012. The rhizosphere microbiome and plant health. Trends in Plant Science, 17, 478–486.
Chen, S., et al. 2018. Plant diversity enhances productivity and soil carbon storage. Proceedings of the National Academy of Sciences, 115, 4027–4032.
Darapuneni, M.K., O.J. Idowu, L.M. Lauriault, S.K. Dodla, K. Pavuluri, S. Ale, K. Grover, and S.V. Angadi. 2019. Tillage and nitrogen rate effects on corn production and residual soil characteristics. Agronomy Journal, 111, 1524–1532.
Diacono, M., and F. Montemurro. 2011. Long-term effects of organic amendments on soil fertility. In E. Lichtfouse, M. Hamelin, M. Navarrete, and P. Debaeke (Eds.), Sustainable Agriculture Volume 2 (pp. 761–786). Dordrecht, The Netherlands: Springer.
Doran, J.W., and M.R. Zeiss. 2000. Soil health and sustainability: Managing the biotic component of soil quality. Applied Soil Ecology, 15, 3–11.
Epstein, E., and A.J. Bloom. 2005. Mineral nutrition of plants: Principles and perspectives, 2nd ed. Sunderland, MA: Sinauer Associates.
Ghimire, R., J.B. Norton, U. Norton, J.P. Ritten, P.D. Stahl, and J.M. Krall. 2013. Long-term farming systems research in the central High Plains. Renewable Agriculture and Food Systems, 28, 183–193.
Ghimire, R., V.R. Thapa, A. Cano, and V. Acosta-Martinez. 2019. Soil organic matter and microbial community responses to semiarid croplands and grasslands management. Applied Soil Ecology, 141, 30–37.
Idowu, O.J., S. Sultana, M. Darapuneni, L. Beck, and R. Steiner. 2019. Short-term conservation tillage effects on corn silage yield and soil quality in an irrigated, arid agroecosystem. Agronomy, 9, 455.
Kremen, C., A. Iles, and C. Bacon. 2012. Diversified farming systems: An agroecological, systems-based alternative to modern industrial agriculture. Ecology and Society, 17, 44.
Li, J., G.S. Okin, L. Alvarez, and H. Epstein. 2007. Quantitative effects of vegetation cover on wind erosion and soil nutrient loss in a desert grassland of southern New Mexico, USA. Biogeochemistry, 85, 317–332.
Magdoff, F., and H. Van Es. 2000. Building soils for better crops. Brentwood, MD: SARE Outreach Publications.
Mulholland, B., and M.A. Fullen. 1991. Cattle trampling and soil compaction on loamy sands. Soil Use and Management, 7, 189–193.
Pellant, M., P.L. Shaver, D.A. Pyke, J.E. Herrick, F.E. Busby, G. Riegel, N. Lepak, E. Kachergis, B.W. Newingham, and D. Toledo. 2018. Interpreting indicators of rangeland health, version 5 [Technical Reference 1734-6]. Denver, CO: U.S. Department of the Interior, Bureau of Land Management, National Operations Center.
Rezaei, S.A., R.J. Gilkes, and S.S. Andrews. 2006. A minimum data set for assessing soil quality in rangelands. Geoderma, 136, 229–234.
Sarwar, M., J.A. Kirkegaard, P.T.W. Wong, and J.M. Desmarchelier. 1998. Biofumigation potential of brassicas. Plant and Soil, 201, 103–112.
Scheu, S. 2002. The soil food web: Structure and perspectives. European Journal of Soil Biology, 38, 11–20.
Stevenson, F.J. 1994. Humus chemistry: Genesis, composition, reactions. Hoboken, NJ: John Wiley & Sons.
Thapa, V.R., R. Ghimire, M.M. Mikha, O.J. Idowu, and M.A. Marsalis. 2018. Land use effects on soil health in semiarid drylands. Agricultural and Environmental Letters, 3, 180022.
UNCCD. 2017. Global land outlook [Online]. Bonn: Secretariat of the United Nations Convention to Combat Desertification. Retrieved August 27, 2019, from https://www.unccd.int/sites/default/files/documents/2017-09/GLO_Full_Report_low_res.pdf
USDA. 2018. 2015 national resources inventory summary report. Washington, D.C.: Natural Resources Conservation Service and Center for Survey Statistics and Methodology, Iowa State University.
Vukicevich, E., T. Lowery, P. Bowen, J.R. Úrbez-Torres, and M. Hart. 2016. Cover crops to increase soil microbial diversity and mitigate decline in perennial agriculture. A review. Agronomy for Sustainable Development, 36, 48.
Resources on Soil Health
- Building Soils for Better Crops, 3rd edition Fred Magdoff and Harold van Es, Sustainable Agriculture Research and Education, 2010 https://www.sare.org/Learning-Center/Books/Building-Soils-for-Better-Crops-3rd-Edition
- A Soil Owner’s Manual: How To Restore and Maintain Soil Health, Jon Sitka CreateSpace Independent Publishing Platform, 2016
- Dirt to Soil: One Family’s Journey into Regenerative Agriculture, Gabe Brown, Chelsea Green Publishing, 2018
- Managing Cover Crops Profitably, 3rd edition, Sustainable Agriculture Research and Education, 2007 https://www.sare.org/Learning-Center/Books/Managing-Cover-Crops-Profitably-3rd-Edition
- Cover Crops, Soil Health Principles and Maximizing Yields (webinars), Kristine Nichols and Ray McCormick, Sustainable Agriculture Research and Education, 2014 https://www.sare.org/Learning-Center/Multimedia/American-Society-of-Agronomy-Cover-Crops-Webinar-Series/Cover-Crops-Soil-Health-Principles-and-Maximizing-Yields
- USDA Natural Resources Conservation Service Soil Health Portal https://www.nrcs.usda.gov/wps/portal/nrcs/main/soils/health/
For Further Reading
CR-656: An Introduction to Soil Salinity and Sodium Issues in New Mexico
https://pubs.nmsu.edu/_circulars/CR656/
CR-672: Understanding and Managing Soil Compaction in Agricultural Fields
https://pubs.nmsu.edu/_circulars/CR672/
CR-676: Interpreting Soil Tests: Unlock the Secrets of Your Soil
https://pubs.nmsu.edu/_circulars/CR676/
CR-687: Managing Organic Matter in Farm and Garden Soils
https://pubs.nmsu.edu/_circulars/CR687/
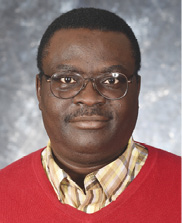
John Idowu is an Extension Agronomist in the Department of Extension Plant Sciences at NMSU. He earned his master's in agronomy from the University of Gottingen in Germany and his Ph.D. in land management from Cranfield University in the UK. His research and Extension activities are focused on sustainable crop production and soil management in New Mexico.
To find more resources for your business, home, or family, visit the College of Agricultural, Consumer and Environmental Sciences on the World Wide Web at pubs.nmsu.edu.
Contents of publications may be freely reproduced, with an appropriate citation, for educational purposes. All other rights reserved. For permission to use publications for other purposes, contact pubs@nmsu.edu or the authors listed on the publication.
New Mexico State University is an equal opportunity/affirmative action employer and educator. NMSU and the U.S. Department of Agriculture cooperating.
December 2019 Las Cruces, NM