Soil Quality of CRP Lands in Eastern New Mexico
Guide A-149
John Idowu and Patrick Kircher
College of Agricultural, Consumer and Environmental Sciences, New Mexico State University
Authors: Respectively, Extension Agronomist, Department of Extension Plant Sciences; and Extension Agriculture Agent, Roosevelt County Cooperative Extension Office, New Mexico State University. (Print friendly PDF)
Introduction
The Conservation Reserve Program (CRP) is a nationwide program for protecting lands that are prone to environmental degradation and loss of wildlife biodiversity. This program was established by the Food Security Act of 1985, in order to protect lands to produce significant benefits to the environment and wildlife conservation (USDA-FSA, 2013a). The ultimate goal was to provide clean air, clean water, soil preservation, and a well-balanced ecosystem through effective stewardship of land.
Subscription to CRP is voluntary, and the federal government offers annual rental payments and cost-share assistance to farmers who are willing to establish long-term resource-conserving ground covers. Farmers' enrollment in the CRP lasts for 10—15 years, with a possible extension of 2—5 years. After the expiration, a farmer is free to use the land for any desired purpose (USDA-FSA, 2013a).
Any of the following criteria must be met before land is enrolled in CRP:
- Highly erodible (easily subject to erosion)
- Contributes to a serious water quality problem
- Provides important wildlife habitat
- Provides substantial environmental benefits if devoted to specific conservation uses
The total CRP land in New Mexico as of March 2013 is 415,644 acres on 1,206 farms (USDA-FSA, 2013b). Acreages that have been retired from CRP between 2006 and 2012 are given on Table 1.
Table 1. Acreages of Land Retiring from CRP from 2006—2012
Year | Acres retired from CRP |
2006 | 7,673 |
2007 | 28,196 |
2008 | 6,039 |
2009 | 29,861 |
2010 | 94,551 |
2011 | 113,058 |
2012 | 71,210 |
USDA-FSA, 2013b |
According to Table 1, large acreages have been exiting the CRP since 2010, and farmers in New Mexico are wondering about the best use for these lands coming out of the CRP. About 65% of CRP lands in New Mexico are located in Roosevelt and Curry Counties in the eastern part of the state (USDA-FSA, 2013c).
Since farmers are allowed to freely use the land exiting the CRP, it is important to develop land-use strategies that will not jeopardize the long-term sustainability of such lands. About 75% of the CRP lands in New Mexico are either in native or newly introduced grasses (Figures 1A and 1B).
Since CRP lands will have been in grasses for at least 10 years, quantifying soil quality in these fields will help farmers make informed decisions on how to use their land after it exits the program.
To answer questions related to soil quality of CRP fields, a study was conducted in Roosevelt County in eastern New Mexico with the objective of quantifying the effects that grasses have had on CRP lands by measuring selected soil quality indicators.
Figure 1A. CRP land in weeping lovegrass.
Figure 1B. CRP land in native grasses.
Choice of Site
Since soil measurements were not taken before the land was converted to CRP, sites where CRP land was adjacent to a farmland within the same soil type were selected for this study. Conventional cropping systems in eastern New Mexico are based on the plow-disk system. This method of farming is normally associated with soil degradation and erosion (Unger et al., 1991). Selected sites, therefore, provided good contrast between conventional farmland and an adjacent CRP land for assessing the effects that perennial grass establishment may have had on soil quality over a long period of time.
A total of eight sites that had been in CRP grasses for at least 15 years were selected for testing. Four of these sites had coarse-textured soils (sandy soils), while the other four had fine-textured soils (clay soils). A CRP field and a farmland field were sampled at each site. Crops grown on the cultivated farmland were cotton, wheat, or sorghum.
Measurements
Soil physical, chemical, and biological measurements were performed either in the field or in the laboratory. Field measurements included soil bulk density and soil penetration resistance. Soil bulk density is an indicator of how densely the soil particles are packed together, which affects soil water movement, water availability for crops, and ease of root growth (Bengough and Mullins, 1990). Soil penetration resistance measures the state of soil compaction. For example, if a soil layer is too compact, the penetration resistance will be very high and roots will not be able to grow through the compacted layer (Bengough and Mullins, 1991).
Bulk density of the soil was measured between 0—6 inches soil depth using a soil core sampler driven into the soil (Figure 2). The soil in the known volume of the sampler was collected into a plastic bag, and the dry mass was determined in the laboratory to compute bulk density. The soil penetration resistance was measured up to a depth of 12 inches using a compaction meter (Figure 3). The compaction meter was gradually pushed through the soil, and the highest value observed on the compaction gauge was recorded as the resistance measurement.
.
Figure 2. Soil bulk density sampler.
Figure 3. Soil compaction meter (or penetrometer).
For many other measurements, representative soil samples were taken at 0—6 inches soil depth from all fields and brought back to the laboratory for analysis.
Another physical measurement conducted on the field soil samples was dry aggregate size distribution, which is measured by placing a known weight of soil on a series of nested sieves, and then shaking the soil with a Ro-Tap sieve shaker for five minutes (Figure 4). The aggregate fractions on each sieve were then collected and weighed. These data were used to calculate three parameters to estimate the susceptibility of the soil to wind and water erosion: mean weight diameter (MWD), aggregates >2 mm (large aggregates), and aggregates <0.25 mm (small aggregates). The higher the MWD and the proportion of the large aggregates, the more the soil will resist erosion. On the other hand, as the small aggregates become more abundant, the soil will be more susceptible to erosion.
Figure 4. Ro-Tap shaker/sieve assembly (A), and sieves (B).
Another measurement performed was biologically active carbon (BAC) using permanganate oxidation technique (Weil et al., 2003). Biologically active carbon has been shown to be a good indicator of soil health. It is well correlated with other soil biological properties such as soil microbial biomass and soil respiration (Weil et al., 2003). Higher BAC values indicate more microbial activity in the soil. The total soil organic matter was also assessed along with many chemical measurements, including nitrate nitrogen, phosphorus, potassium, pH, electrical conductivity, and sodium adsorption ratio.
Results
Soil Physical Measurements
Bulk Density and Penetration Resistance
From Figures 5A and 5B, the bulk density and penetration resistance were significantly lower in the CRP land compared to the farmland. The bulk density was also significantly lower in clay soil compared to sandy soil, but the penetration resistance was not different between clay and sandy soils.
This result shows that the grasses have softened the soil and provided a more conducive environment for roots and water to penetrate. The penetration resistance measured at 12 inches on the farmland was very hard (Figure 5B) and has already exceeded the threshold of 2 megapascals (MPa), which is regarded as too compact for agricultural crops (Hamza and Anderson, 2005).
The reduction in soil density and penetration resistance in the CRP land may be related to the extensive network of grass roots that have penetrated the soil for a long period of time. In addition, the sandy soils exhibited a higher density than the clay soils, which indicates that clay soils on these CRP lands will allow better water movement and rooting in the surface soil compared to the sandy CRP soils in eastern New Mexico.
Figure 5A. Bulk density of the CRP and farmland in soils of different textures.
Figure 5B. Penetration resistance of the CRP and farmland in soils of different textures.
Aggregate Size Distribution
No difference was seen in the MWD or proportion of large and small soil aggregates between the CRP and farmland (Figures 6A—6C). The only difference observed was due to texture. Based on this result, the sandy soils will experience more wind erosion compared to the clay soils since they had lower MWD and lower amounts of large aggregates (Figures 6A and 6B). Since the CRP and farmland did not differ with respect to the dry aggregate size distribution, both of them will erode equally if there is no protective vegetation cover.
Figures 6A—6C. Mean weight diameter (A), large aggregates (B), and small aggregates (C) in the CRP and farmland under different soil textures.
Soil Biological Measurements
Biologically Active Carbon and Total Soil Organic Matter
There was no significant difference between the CRP and farmland for BAC (Figure 7A).
The only difference observed was a significantly higher BAC in clay soils compared to sandy soils. We also observed that the CRP soil had higher soil organic matter than the farmland in the clay soils; however, there was no difference between them in the sandy soils (Figure 7B). It is also worth noting that the soil organic matter was very low in the sandy soil (<0.7%) for both the CRP and the farmland. This indicates the need for great caution concerning sandy soils exiting the CRP. Such soils are still fragile and need to be carefully managed if they are to be converted to farmland.
Figure 7A. Biologically active carbon of the CRP and farmland in soils of different textures.
Figure 7B. Soil organic matter of the CRP and farmland in soils of different textures.
Soil Chemical Measurements
There were no significant differences in the chemical measurements that were assessed in this study (Table 2). The soil salinity was low, and there was also no sodium problem on both the CRP and farmlands.
Table 2. Soil Chemical Measurement Values of the CRP and Farmland Soils
Clay | Sand | Level of significance |
|||
CRP | Farm | CRP | Farm | ||
Nitrate nitrogen (ppm) | 3.3 | 12.9 | 4.0 | 7.6 | NS |
Potassium (ppm) | 50.3 | 58.5 | 44.3 | 56.5 | NS |
Extractable phosphorus (ppm) | 6.8 | 15.6 | 7.2 | 10.4 | NS |
Electrical conductivity (dS/m) | 0.33 | 0.61 | 0.31 | 0.40 | NS |
pH | 7.55 | 7.25 | 7.33 | 7.28 | NS |
Sodium adsorption ratio | 0.13 | 0.15 | 0.14 | 0.11 | NS |
NS: Not significant |
Significance of Results
These results present preliminary insight into the relative gains in soil health of the CRP grasslands in dryland, semi-arid ecosystems of eastern New Mexico. The information presented here is meant to guide farmers and stakeholders toward making appropriate decisions regarding the utility of lands exiting the CRP program in eastern New Mexico. Important findings include:
i) | Despite the long-term, permanent grasses of the sandy CRP fields, there has been no appreciable accumulation of organic matter in the CRP land—especially in the sandy soils—when compared to the adjacent farmlands. For clay soils in CRP, there was a small increase of about 21% in the soil organic matter compared to the farmed soils. Soil organic matter consists of plant and animal residues at different stages of decay in the soil, which stores nutrients and water in the soil, helps reduce soil compaction, and increases water infiltration. The higher the soil organic matter, the better the soil's capacity to support crop production. Therefore, since the sandy CRP soils have not experienced significant increases in organic matter compared to adjacent farmlands, the capacity of sandy soils exiting the CRP to support crop production will be limited. These sandy soils may require higher levels of inputs for crop production compared to the fine-textured CRP soils, which had experienced some organic matter increase. |
ii) | Erosion potential of the soil has not been significantly reduced by the long-term, permanent grasses, as shown by the dry aggregate size distribution. This means that CRP soils will erode just as much as farmland soils if both of them are bare. It is therefore advisable to keep the ground covered to prevent accelerated erosion by wind and/or water. |
Some suggested uses for lands coming out of the CRP include:
- Rangeland: Lands coming out of the CRP can be maintained as grazing land, but be careful to not overgraze to avoid destroying the regrowth potential of the grasses.
- Hay production: Due to a high demand for forages, it is possible to cut and bale the grasses from these fields, especially weeping lovegrass. It is important to not cut the grasses too low in order to maintain future regrowth potential.
- If crop production is being considered, the best option is to use a non-inversion tillage method for land preparation. Since the CRP soils do not have compaction problems (as shown by the low bulk density and penetration resistance), no-till or strip-till systems can be used for row-crop production. These reduced tillage methods will ensure a protective residue on the soil surface and promote better soil moisture conservation.
- Adding manure, compost, or other organic amendments will benefit lands coming out of CRP, especially if crop production is being considered. These organic amendments can help build up the soil organic matter, which will lead to a better soil structure capable of withstanding erosion.
Conclusion
Since large acreages of land recently came out of the CRP in New Mexico and many more will be coming out in the next few years, it is important for farmers to be aware of the quality of their land after an extended period in grasses. Results from this study indicate that the CRP lands in eastern New Mexico have not improved much with respect to critical soil quality indicators, such as soil organic matter and erosion potential. Sandy CRP fields had the lowest soil quality, with no significant effect of long-term grasses on the soil organic matter compared to farmlands nearby. The fine-textured CRP lands had a small increase (21%) in the soil organic matter compared to farmlands. The soil erosion potential was not different between the CRP and the farmland. This shows that these soils are still very fragile and need to be carefully managed to prevent rapid erosion and degradation. Farmers are encouraged to use the land in ways that maintain plant cover on the soil at all times and to add organic amendments if available.
References
Bengough, A.G., and C.E. Mullins. 1990. Mechanical impedance to root growth: A review of experimental techniques and root growth responses. Journal of Soil Science, 41, 341—358.
Bengough, A.G., and C.E. Mullins. 1991. Penetrometer resistance, root penetration resistance and root elongation rate in two sandy loam soils. Plant and Soil, 131, 59—66.
Hamza, M.A., and W.K. Anderson. 2005. Soil compaction in cropping systems: A review of the nature, causes and possible solutions. Soil and Tillage Research, 82, 121—145
Unger, P.W., B.A. Stewart, J.F. Parr, and R.P. Singh. 1991. Crop residue management and tillage methods for conserving soil and water in semi-arid regions. Soil and Tillage Research, 20, 219—240.
USDA-FSA. 2013a. Conservation Reserve Program [Online]. United States Department of Agriculture Farm Service Agency. Retrieved May 8, 2013 from, https://www.fsa.usda.gov/Internet/FSA_File/crpfactsheet0213.pdf
USDA-FSA. 2013b. Conservation Reserve Program monthly summary — March 2013 [Online]. United States Department of Agriculture Farm Service Agency. Retrieved May 8, 2013, from https://www.fsa.usda.gov/Internet/FSA_File/mar2013stat.pdf
USDA-FSA. 2013c. Change in crop acreage, from September 30, 2012 to October 31, 2012 [Online]. United States Department of Agriculture Farm Service Agency. Retrieved May 8, 2013, from https://www.fsa.usda.gov/Internet/FSA_File/acrescounty012213.xls
Weil, R.R., K.R. Islam, M.A. Stine, J.B. Gruver, and S.E. Samson-Liebig. 2003. Estimating active carbon for soil quality assessment: A simplified method for laboratory and field use. American Journal of Alternative Agriculture, 18, 3—17.
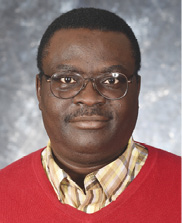
John Idowu is an Extension Agronomist in the Department of Extension Plant Sciences at NMSU. He earned his master's in agronomy from the University of Gottingen in Germany and his Ph.D. in land management from Cranfield University in the UK. His research and Extension activities are focused on sustainable crop production and soil management in New Mexico.
To find more resources for your business, home, or family, visit the College of Agricultural, Consumer and Environmental Sciences on the World Wide Web at pubs.nmsu.edu
Contents of publications may be freely reproduced for educational purposes. All other rights reserved. For permission to use publications for other purposes, contact pubs@nmsu.edu or the authors listed on the publication.
New Mexico State University is an equal opportunity/affirmative action employer and educator. NMSU and the U.S. Department of Agriculture cooperating.
May 2014